Converting power from high voltage sources is very challenging, especially because you need to design the isolation as well as the transformer. Hi, I'm Surinder Singh. I'll show you how WEBENCH tools can help you overcome these challenges. Let us take an example from everyday life. I need to charge the handheld device, but the power that is available to me is coming from the wall at an AC voltage, which is typically a very high voltage. In this scenario, what are my key requirements? Of course, I need to make sure that my design balances efficiency, footprint, and cost, and, of course, I'm able to regulate to the desired DC level, and, very importantly, I am able to provide electrical isolation. When there is no direct conduction path from the high voltage input side to the low voltage DC side, we say that a power converter is isolated. And isolation is important because if the electrical shorts or component failures or surges, they are not transmitted to the other side. And this protects both the human operator as well as the equipment. One of the most popular topologies for converting power from high voltages is the flyback topology. Central to the idea of the operation of the flyback is the flyback transformer. And the flyback transformer provides both isolation, as well as it provides an inductor for storage of the energy. And this makes a topology a very, very popular topology. Let's take a look at the operation of the flyback converter. The flyback transformer on the primary side is connected to a semiconductor switch. On the secondary side, there is a diode, which provides the rectification function. The primary is directly connected to a DC source. But if the input to our converter is not a DC but an AC, we can convert it into DC using full-bridge rectification. Now, let's take a look at how the flyback operates. When the MOSFET is on, the DC source is directly connected to the primary side. Hence, the current in the primary will keep increasing. This increasing current will store the energy into the magnetic core. And in this stage, the output diode is not conducting because it is reverse-biased. When the MOSFET turns off, the same energy which is just stored in the magnetic core is now transferred into the secondary side and the diode forward biases. Sometimes, we have a third stage of operation, in which the magnetic energy goes to zero. And this stage is called the Discontinuous Mode, or the DCM mode. A typical flyback converter also has other components. We talked about the AC/DC full-bridge rectifier. But there is a control IC, which has to drive the external MOSFET. And because they could be ringing on the drain of the MOSFET, you need to design these numbers. And of course, from the output side, we need to regulate the voltage. So some sensing mechanism has to happen, which is usually through an optocoupler, which provides the feedback back to the control IC. This control IC, of course, needs power too, so this power is sometimes generated from the same transformer using auxiliary windings. The flyback transformer, as you can see now, is the core of how the flyback works. And unlike the transformer in other scenarios, this transformer is really an coupled inductor. So it's stores the energy during one phase and delivers it to the output in the other phase. The flyback is a complex topology to design. The biggest challenge lies in designing the flyback transformer itself. The designer must choose from a large variety of cores and bobbins available and at the same time making sure that the electrical targets are satisfied and the other constraints are respected. So let's look at some of these targets and constraints in more detail. The primary inductance, which determines the ripple current, needs to be of a certain value. And this depends on the turns ratio, which is a design parameter, and the core cross-section area and the air gap. So if the number of turns increase, the primary inductance also increases. The magnetic material itself goes through a process called saturation. So we need to make sure that our design is such, which means that our maximum current and the number of turns is such that we do not hit the saturation. In fact, we stay away from it and set a target of a Bmax. Next, we need to make sure that the power dissipated into the copper wiring is less than a certain target value. This power itself is estimated by knowing what the DC resistance of the copper wire itself is. And as shown in this formula, this is proportional to the connectivity of the copper and the length of the copper wire. The length of the copper wire itself depends on the number of turns and the mean length per turn, which is a property of the core. A loss constraint is really a physical constraint, which says that the area available inside the core must be utilized such that all the wiring can fit into there. And since there is a certain amount of space loss, because of insulation and the circular shape of the wire, the fill factor cannot completely be 1. So its typically between 0.5 and 0.9. From these four constraints, we can eliminate the design unknowns of number of turns, air gap, and wire cross section and express it in the form of the following inequality. The left side depends only on the physical dimensions of the core. And it is called the core geometrical constant, Kg. Its units are length to the power of fifth. The right hand side depends on the property of the design, which is the electrical and the magnetic properties. This inequality is very powerful, because it tells us how to select the cores. That means, the core Kg must be larger than the design Kg. For example, if we need to have larger amount of current in our design, which means that the required Kg from the cores would shoot up. Now, we take all these constraints, and we can design the rest of the transformer. So we can now determine the number of turns in the primary. And based on the turns ratio, we can set the number of turns in the secondary and the auxiliary. And we can also specify the so-called inductance factor, which we have so far expressed in terms of the air gap. And at this point, our transformer is ready to be designed. This transformer design algorithm, if done manually, is not only laborious and time-consuming to do but will also give suboptimal results. Here, the WEBENCH Power design tools can be of great help. So let's see how we can create designs using very WEBENCH tools. Let us design with the UCC28C42. So go to the product folder of this page, where you will find a WEBENCH panel on which you can put in your design input/output conditions. Click on Open Design. And in a few seconds, you'll be presented with the entire design done in the form of a summary. You'll see your schematic, your bill of materials, operating values presented to you. The power loss contributions are automatically calculated. And you can view them in charts. And you can view the other operating values in the Operating Values tab also. The bill of materials can be downloaded from the Bill of Materials tab. And you can print out the PDF of your entire design, as well as share it with your coworkers. Now, let's look at the transformer that the tool created for us. So click on the schematic, where you'll see the symbol for the transformer. Click on it, and it will open up a window showing you the transformer that the tool designed for you. In this window, you can see the core part number that has been selected, the bobbin, as well as all designing information and the electrical characteristics that the tool computed for you. If you're happy with this, you can continue your design with this transformer. But if you wanted to explore more cool bobbin combinations, click here, which will take you to this page. Here, you can look at other combinations of cores and bobbins, which will work for your design, and the key characteristics of those cores and bobbins. And specifically, if you wanted to look at Kg, the geometrical factor, you can look at here. Now, let's do something interesting. Let's change the design conditions from 2 amps to 1 amp. So we're basically reducing the amount of power that we need by about 1/2. So go back to the schematic, click on the Explore the Core Bobbin Combination. And you go back and look at the Kg's. So now, you'll find that the Kg value is less than what we had required for a 2-amp design. A more closer comparison also reveals that the winding ratios are different, the wires used are different, and the primary inductances that the tool calculated are different for the 2-amp and the 1-amp design. You'll also notice something very interesting, that the 1-amp design results in transformers which are cheaper, have a smaller footprint, and a smaller Kg. This is all what we expected. But the tool has done it for us. Once you are satisfied with your design, go back to the transformer design page and download the transformer report. This PDF report contains all the information that you need to manufacture your transformer-- so things like number of turns, winding diagrams, core and bobbin part numbers. You can handle this report to your manufacturing department and get your transformer fabricated. So what we have seen is, the WEBENCH design tools in a matter of minutes can take your input/output specifications down to a report for your transformer. I enjoyed giving this presentation. Please explore WEBENCH transformer designer for your next isolated and high voltage design. Thank you.
I need an excel sheet for the transformer differential protection relay RET615. The input will be the relay settings, and it should be capable of calculating Ida, Idb, Idc and Ira, Irb, Irc under any combination of injected phase currents, for any vector group of the transformer. This spreadsheet completes the design of single pad foundations including a central column. The column can include axial, horizontal and moment forces. The spreadsheet includes a simplified version for fast calculation of straightforward pad foundations. Eccentric Footing Design Excel Sheet. The UCC25600 Design Tool is an Excel Spreadsheet based calculation sheet to help design an LLC half bridge converter using UCC25600. The tool helps design the power stage and program the IC setup parameters. The power stage design includes resonant tank, primary and secondary switches and magnetic elements.
Description
Design your isolated high-voltage power supply as well as the transformer. In this video we will take you through the design process of designing an isolated AC/DC or DC/DC power supply. We will highlight the key design constraints for designing the transformer and show you how the WEBENCH tool designs the transformer for you. Using this video, for example, you will be easily be able to design a low-voltage DC power for a handheld device from a high-voltage AC power socket using the Flyback topology, while maintaining low cost, high efficiency and small size.
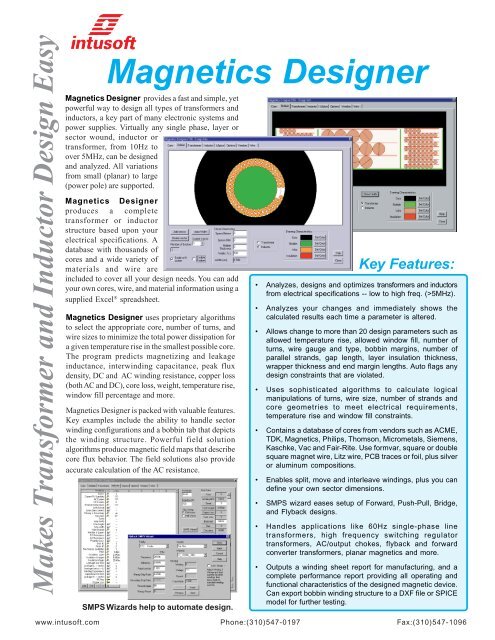
Key take-aways:
- Transformer Design Calculation Excel 8/26/2019 We've built a transformer tank to house the core and this will be filled with oil, a radiator was built as well - I have to do thermal prediction calculations and am at a complete loss.
- This application is designed for design engineers working in transformer industry. For more information please visit www.rentec.in.
Learn why high voltage power supplies need isolation and how it is implemented
Understand the operation and key advantages of the popular Flyback topology
Get insight into the design constraints of the Flyback transformer (FBT), or the coupled Inductor, and see how the WEBENCH® Designer designs the transformer with these constraints
Learn how to use WEBENCH® Designer to design an isolated, high-voltage power supply and its transformer
Walk through an exercise using the UCC28C42 BiCMOS current-mode PWM controller
PDFs for download
Additional information
Transformer Design Calculation Excel Template
Downloads
Supported products & hardware
Boost controllers (external switch)
LM3017—High Efficiency Low-Side Controller with True ShutdownLM3478—2.97~40V Wide Input Range Boost/SEPIC/Flyback DC-DC ControllerLM3478Q-Q1—40-V wide VIN low-side N-channel controller for switching regulators with 10-µA shutdown currentLM3481—2.97V to 48V High-Efficiency Controller for Boost, SEPIC and FlybackLM3481-Q1—2.97-V to 48-V high-efficiency controller for boost, SEPIC and flyback, AEC-Q100 qualifiedLM3488—2.97V to 40V Wide Vin Low-Side N-Channel Controller for Switching RegulatorsLM3488Q-Q1—40-V wide VIN low-side N-channel controller for switching regulators with 5-µA shutdown currentLM5020—13-100V Wide Vin, Current Mode PWM Boost, Flyback, Sepic ControllerLM5022—6-60V Wide Vin, current mode boost, SEPIC and Flyback controllerTPS40210-Q1—4.5-V to 52-V wide input range current mode boost controller with 700-mV reference voltageTPS40211-Q1—4.5-V to 52-V wide input range current mode boost controller with 260-mV reference voltageBoost converters (integrated switch)
LM2577—3.5-V to 40-V, 3-A low component count step-up regulatorLM2585—4-V to 40-V, 3-A step-up wide VIN flyback regulatorLM2586—4-V to 40-V, 3-A step-up wide VIN flyback regulator with frequency synchronizationLM2587—4V to 40V, 5A Step-Up Wide Vin Flyback RegulatorLM2588—4-V to 40-V, 5-A step-up wide VIN flyback regulator with frequency synchronizationLM2621—1.2V to 14V input voltage, 1A load current, step-up DC-DCLM2623—0.8V to 14V input voltage, 2A load current, step-up DC-DCLM2623-Q1—0.8-V to 14-V input voltage, 2-A load current, step-up DC/DC, AEC-Q100 qualifiedLM2735—520kHz/1.6MHz space efficient boost and SEPIC DC-DC regulatorLM2735-Q1—520-kHz, 1.6-MHz space efficient boost and SEPIC DC/DC regulator, AEC-Q100 qualifiedLM3578A—2-V to 40-VIN switching regulators in buck, boost, terminal, depending upon the applicationLM5000—3.1-40V Wide Vin, 2A Current Mode Non-Synchronous Boost RegulatorLM5001—3.1-75V Wide Vin, 1A Current Mode Non-Synchronous Switch Mode RegulatorLM5001-Q1—3.1-V to 75-V wide VIN, 1-A current mode non-synchronous switch mode regulatorLM5002—3.1-75V Wide Vin, 0.5A Current Mode Boost, Flyback, Sepic ConverterTL1451—Dual Pulse-Width-Modulation Control CircuitTL1451A—3.6V to 50V dual channel controller with Wide input voltage rangeTL1451A-EP—Enhanced Product Dual Pulse-Width-Modulation Control CircuitsTL1451A-Q1—3.6-V to 50-V dual-channel PWM controller, AEC-Q100 qualifiedTL5001—PWM Controller with wide input range, with ±5% tolerance on reference, operation –20°C to 85°CTL5001A—PWM Controller with wide input range, with ±3% tolerance on reference, operation –20°C to 85°CTL5001A-Q1—PWM Controller with wide input range, with ±3% tolerance on reference, operation –20°C to 85°CTL5001M—PWM Controller with wide input range, with ±5% tolerance on reference, operation –55°C to 125°CTPS43000—Multi-Topology (buck, boost, sepic) High Frequency DC/DC ControllerTPS55340—5-A, 40-V, wide VIN boost, flyback, & SEPIC converterTPS55340-EP—Enhanced integrated, 5-A, 40-V wide input range boost/SEPIC/flyback DC/DC regulatorTPS55340-Q1—Integrated, 5-A 40-V wide input range boost/SEPIC/flyback DC/DC regulator, AEC-Q100 qualifiedTPS61175—3-A ,40V High Voltage Boost Converter with Soft-start and Programmable Switching FrequencyTPS61175-Q1—3-A, 40-V high voltage boost converter with soft-start and programmable switching frequencyUC2572—Negative output Flyback pulse width modulator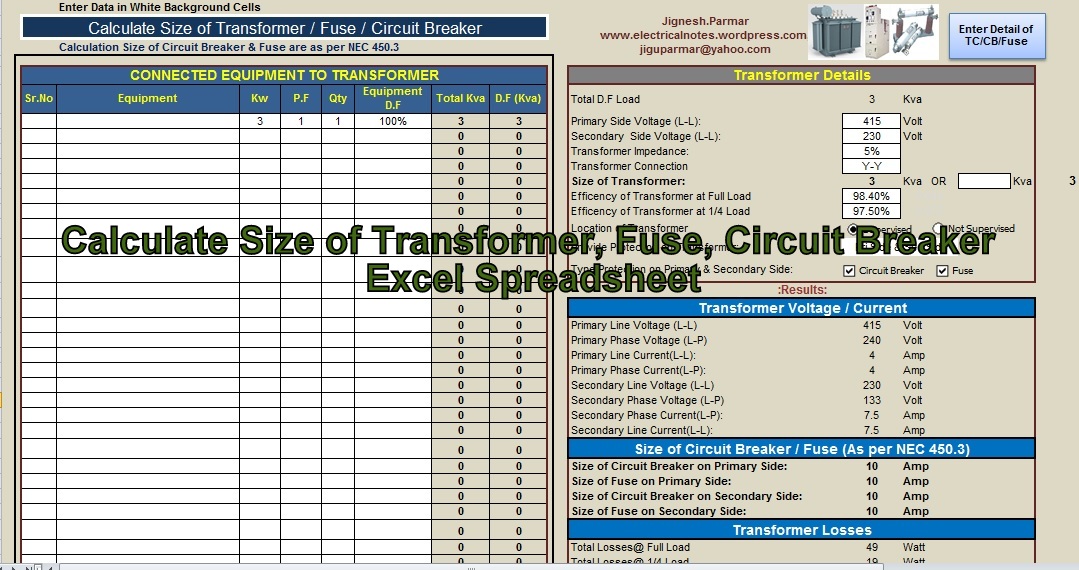
Buck converters (integrated switch)
LM5015—4.25-75V, Monolithic Two-Switch Forward DC-DC RegulatorFlybuck converters
LM25017—7.5-48V Wide Vin, 650mA Constant On-Time Synchronous Buck RegulatorLM25018—7.5-48V Wide Vin, 300mA Constant On-Time Synchronous Buck RegulatorLM25019—7.5-48V Wide Vin, 100mA Constant On-Time Synchronous Buck RegulatorLM34925—7.5-100V Wide Vin, 100mA Integrated Secondary Bias Regulator for Isolated DC/DC ConvertersLM34926—7.5-100V Wide Vin, 300mA Integrated Secondary Bias Regulator for Isolated DC/DC ConvertersLM34927—7.5-100V Wide Vin, 600mA Integrated Secondary Bias Regulator for Isolated DC/DC ConvertersLM5017—7.5-100V Wide Vin, 600mA Constant On-Time Synchronous Buck RegulatorLM5018—7.5-100V Wide Vin, 300mA Constant On-Time Synchronous Buck RegulatorLM5019—7.5-100V Wide Vin, 100mA Constant On-Time Synchronous Buck RegulatorLM5160—LM5160 wide input 65V, 2A synchronous buck/Fly-Buck™ converterLM5160-Q1—Wide Input 65V, 2A Synchronous Buck / Fly-Buck™ ConverterLM5160A—LM5160A Wide Input 65V, 2A Synchronous Buck / Fly-Buck™ ConverterLM5161—Wide Input 4.5-V to 100-V, 1-A Synchronous Buck / Fly-Buck ConverterTPS55010—2.95V To 6V Input, 2W, Isolated DC/DC Converter with Integrated FETSTransformer Design Calculator
PWM controllers & resonant controllers
LM2524D—40V, 0.2A 550KHz PWM controllerLM3524D—40V, 0.2A 350KHz PWM controller